Testing Turing's Hypothesis
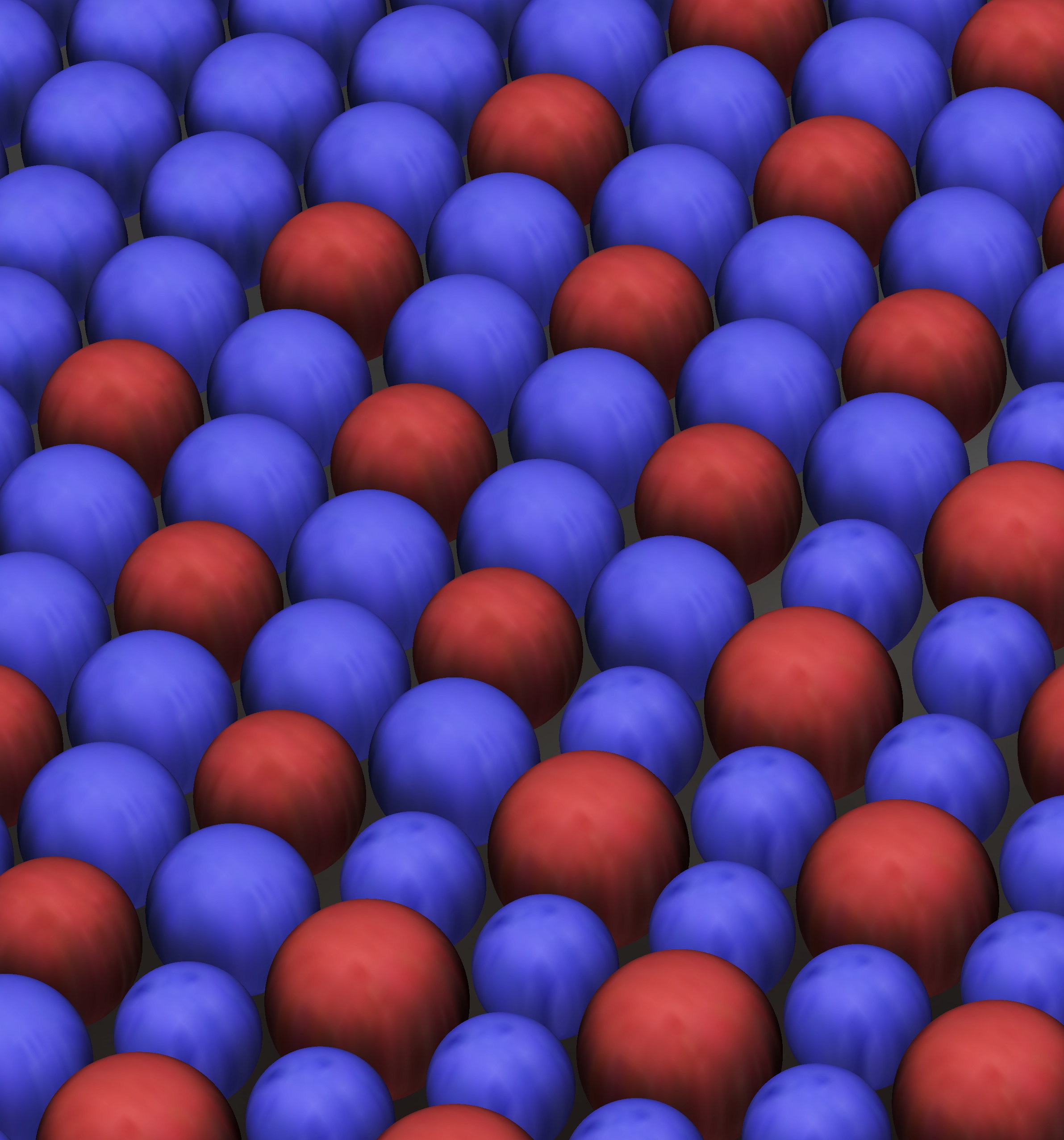
Some popular press about our work
Interview in Engineering and Technology Magazine in an issue dedicated to Turing.
Radio Interview I with Irv Epstein on WCAI.
Father of Computer Science Also Left a Mark on Biology
Radio Interview II
Read the transcript and listen to Seth and Irv discuss our paper, Testing Turing, during an interview on RadioBoston (WBUR, Boston's NPR news station).
Portuguese description of our PNAS paper in O ANO DA VIRADA DE TURING. Source GAZETA DE MATEMÁTICA
Introduction
Testing Turing's Theory of Morphogenesis in Chemical Cells
Nathan Tompkins, Ning Li, Camille Girabawe, Michael Heymann, G. Bard Ermentrout, Irving R. Epstein, and Seth Fraden
Proceedings of the National Academy of Sciences 111, 4397–4402, 2014.
Alan Turing, in his paper The Chemical Basis of Morphogenesis described how, in circular arrays of identical biological cells, diffusion can interact with chemical reactions to generate up to six periodic spatiotemporal chemical structures. Turing proposed that one of these structures, a stationary pattern with a chemically determined wavelength, is responsible for differentiation. We quantitatively test Turing’s ideas in a cellular chemical system consisting of an emulsion of aqueous droplets containing the Belousov-Zhabotinsky (BZ) oscillatory chemical reactants, dispersed in oil, and demonstrate that reaction-diffusion processes lead to chemical differentiation, which drives physical morphogenesis in chemical cells. We observe five of the six structures predicted by Turing. In 2D hexagonal arrays, a seventh structure emerges, incompatible with Turing’s original model, which we explain by modifying the theory to include heterogeneity.
A dish and stirred beaker of BZ are shown below. Zhabotinsky worked at Brandeis from 1991 until his death in 2008. An article by Winfree describes the interesting story behind the BZ reaction. Zhabotinsky's obituary in Nature was written by our collaborator Irv Epstein. In these videos the dish of BZ shows travelling waves whereas the stirred beaker uniformly changes color.
Chemical Cells
We create chemical cells to model biological cells by creating an aqueous emulsion within a fluorinated oil stabilized by surfactants using a standard procedure known as "flow focusing". The video below shows two streams of BZ reactants being mixed on a PDMS chip and then flow-focused into an emulsion. Each drop is small enough to be uniform on the time scale of the reaction due to diffusion alone so each drop acts like the beaker from above.
We can then pack the BZ emulsion into a thin glass capillary and observe their collective behavior. The video below shows a zoomed in region of a large collection of drops. The drops synchronize into a 120 degree oscillation pattern where for every triangle of drops the three members take turns oscillating with a 120 degree phase difference. These triangles have a spontaneous symmetry of right and left handed oscillation patterns.
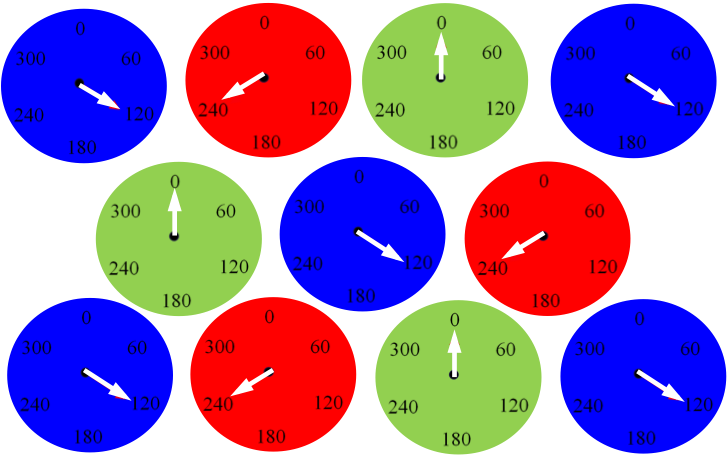
Looking at the patterns of rings of coupled oscillators leads to different behavior in rings with an even or odd number of members. Below is a video from the 2012 IGERT poster and video competition (we won the judges choice award) that describes some of the theory and experiments.
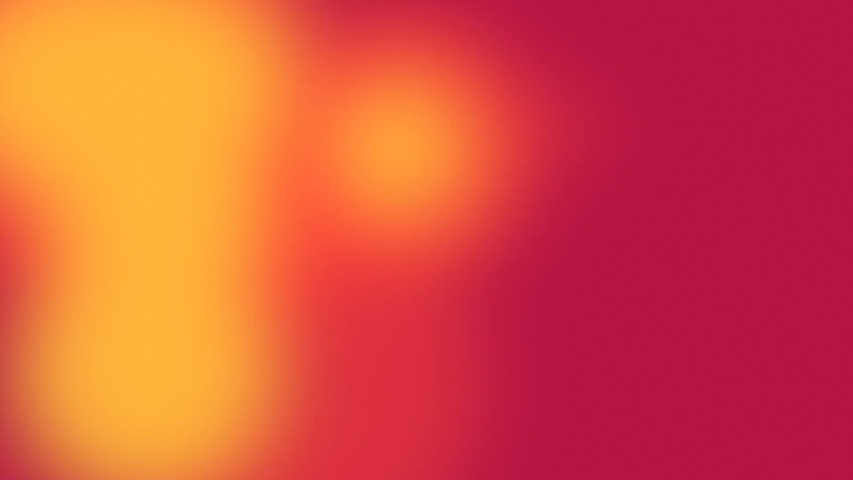
Another way of analyzing the data is to look at a space-time plot where a single line of pixels from a video is plotted as an image. The video below demonstrates a space-tome plot being created from a line of oscillating drops packed in a capillary. In this video we've used light to set all of the drops initially in-phase and the video watches as they assume an anti-phase pattern.
Optical isolation of chemical oscillators
With the BZ emulsion we can now use our Programmable Illumination Microscope (PIM) to optically isolate individual chemical oscillators. The video below demonstrates this.
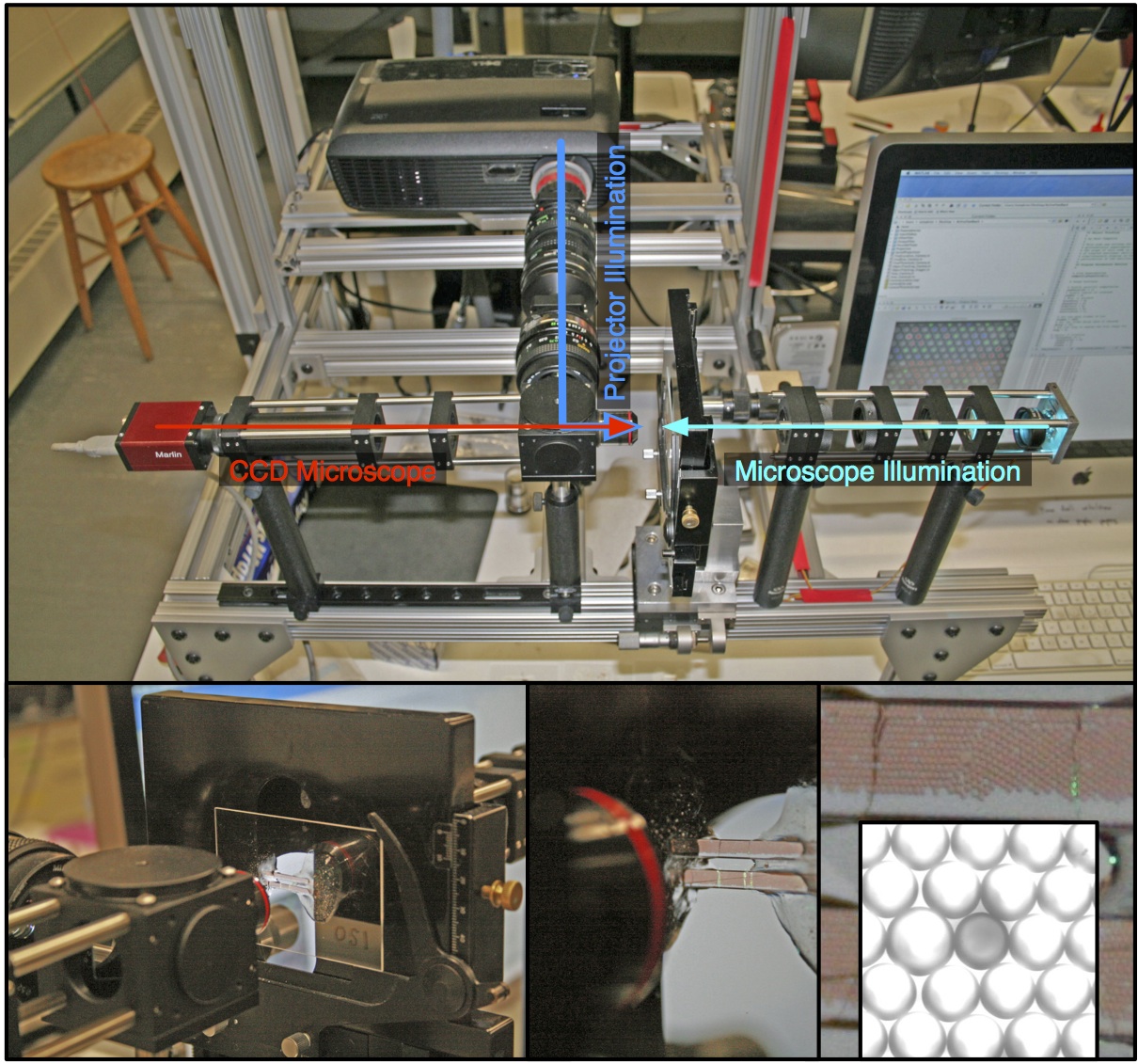
In the first third of the video, the drops oscillate uninhibited and adopt a pattern where nearest neighbors are 120 degrees out of phase with each other. Then (invisible) light is shone on all drops but the center one, which suppresses the oscillations in the illuminated drops. In the final third of the video the light is turned off and all drops resume oscillations.
Using the optical isolation capabilities demonstrated above boundary conditions can be imposed to create one-dimensional rings of drops. These geometries of rings are very useful to be able to study experimentally as they reproduce the periodic boundary conditions often studied theoretically and in simulations. Allan Turing's 1952 paper "On the Chemical Basis of Morphogenesis" is one example of a theoretical paper we can study experimenally with this technique. Our recently published paper in PNAS specifically tests Turing's hypothesis. All of the supplemental videos are available from PNAS and from the publications section of our website. One video is shown below. In this video rings of drops with five and six members are optically isolated from a close-packed hexagonal array of drops. The only difference between the two rings is the number of drops and that is enough to control the synchronization pattern observed.
Belousov-Zhabotinsky Dynamics
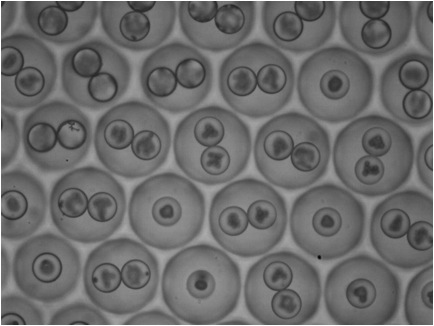
-
Using the BZ chemistry and microfluidic techniques we also study the dynamics of BZ in an emulsion environment.
Double Emulsions
One avenue of study is to look at the effects of BZ in a double emulsion environment (an emulsion within an emulsion).
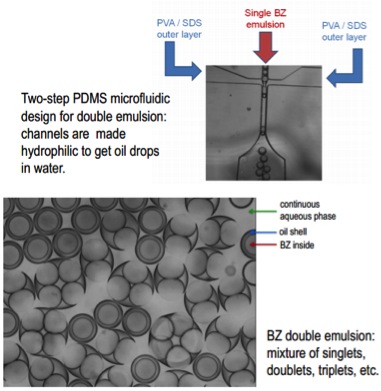
Chemical Conditions
Another avenue of study is to look at the effects of different chemical conditions within an emulsion environment. The videos below demonstrate the dynamics and high and low malonic acid (MA) concentration. At high MA a single wave travels across the emulsion and smaller drops are then stationary while larger drops oscillate.
At low MA large drops still oscillate but smaller drops form a pattern of some bright some dark that slowly changes with time.
Active Matter
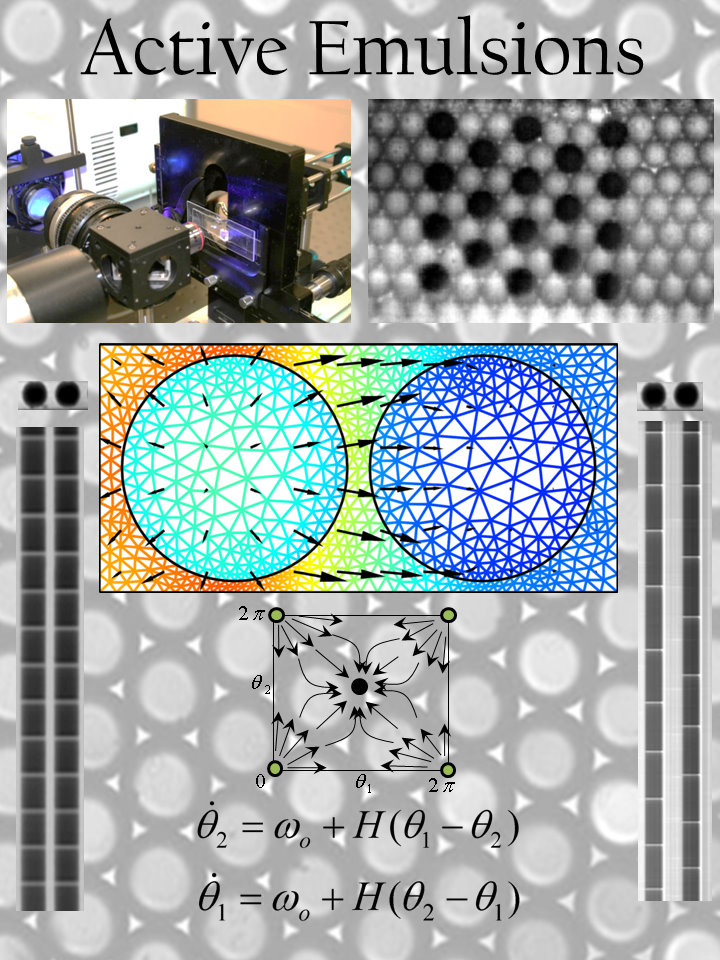
Active Matter: Interacting Nonlinear Chemical Oscillators (Funded by the Brandeis MRSEC) Joint research with Bing Xu (chem), Irv Epstein (chem), Bulbul Chakraborty (physics).
Living matter possesses remarkable material properties, such as the ability to sense the environment, make decisions based on sensory input, and through self-propulsion, move in the direction of choice. The simplest single cellular organism, the bacterium, possesses these properties, as do many multi-cellular organisms. Some bacteria and amoeba, such as Dictyostelium exhibit chemotaxis – or navigate along chemical gradients to forage for food. In other circumstances, such as starvation, individual Dictyostelium communicate with each other through a chemical reaction-diffusion mechanism and self-organize into a kind of tissue where the single cells aggregate into a fruiting body for survival. Some bacteria aggregate and then protect themselves by enveloping themselves in a starch coat called a bio-film. These materials have the ability to heal themselves. The goal of this project is to create a new class of materials, which have properties heretofore only associated with living matter. Like living matter, these materials will be active in the sense that they must consume energy in order to function, but will be entirely synthetic with no biological components at all. Combining methods of experiment, simulation and theory and personnel spanning the disciplines of neuroscience, computer science, physics and chemistry we will lay the scientific framework to create materials that have properties like trees that grow thicker and stronger in response to stress imposed by wind or snow, or muscle tissue, which increases in response to exercise.
The materials we will study are based on the Belousov-Zhabotinsky chemical reaction. The BZ system produces an oscillating redox reaction of a metal ion. In thermodynamically closed systems the BZ oscillations can occur 100 times, but will last forever in an open system in which the BZ reactants are continuously fed into the solution. In analogy with living matter, we produce “cells” by isolating the aqueous BZ reagents in either gels or in emulsion droplets where oil forms the continuous phase. We combine individual cells to create a “tissue” composed of many cells separated by a matrix or fluid.
A limited subset of the aqueous BZ chemicals permeates through the hydrophobic intercellular medium giving rise to intercellular communication. We also introduce light sensitive catalysts that allow external control of the reaction. We fabricate cells between 10 microns and 200 microns in diameter. Using a computer programmable illumination system we can individually “address” each cell and control the phase of the chemical oscillator using light. When confined to a two dimensional layer we can simultaneously image and control about 1000 cells.
The BZ chemistry is complex and can be understood semi-quantitatively at a detailed level of 80 chemical reactions. Focusing on the rate limiting reactions allows a simplified description in terms of two species; an activator and an inhibitor, which interact in three distinct steps. First, the metal ion is in the reduced state. The inhibitor suppresses oxidation of the metal ion while a slow process consumes the inhibitor. Second, once the inhibitor falls below a critical threshold, the activator is unleashed and an autocatylic reaction leads to a rapid oxidation transition consuming all the reduced metal ion. Third, once the oxidation is complete a slow process reduces the oxidized metal ion while simultaneously producing the inhibitor, thus restarting the oscillation.
Check out our BZ Bootcamp 2011.